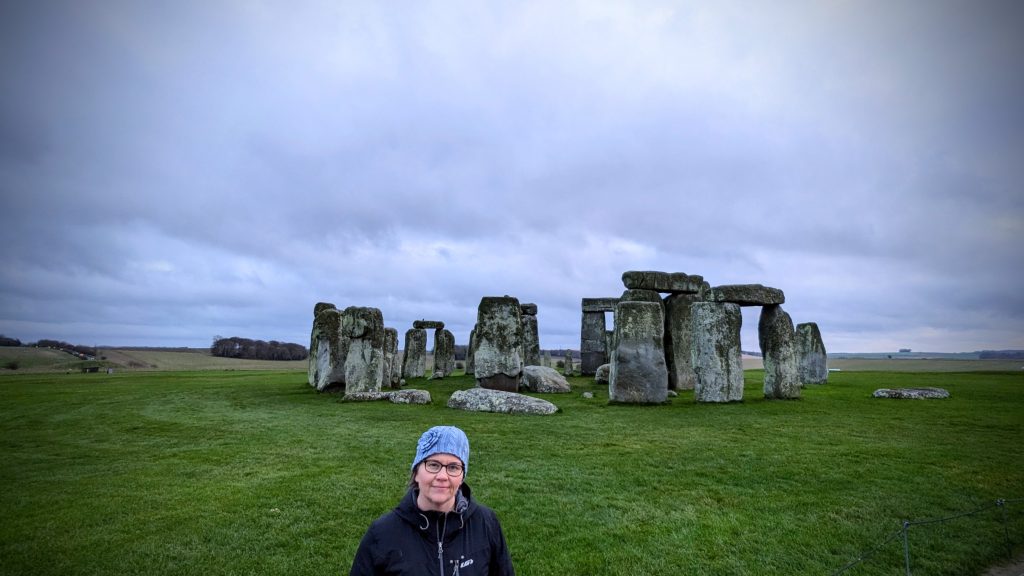
(Photo provided)
Citizen Science #24 by Jamie Zvirzdin
Energy Demystified: Honoring Light from Stonehenge to Science
Mere days before the winter solstice this year, on December 17th, I had the opportunity to visit Stonehenge with my son and husband. Driving clockwise in roundabouts and on the left-hand side of narrow roads gave my brain a workout, but I could still spare a moment of shock as I crested the top of a hill and saw the lone group of large stones surrounded by fields and sheep. As we walked around Stonehenge, we marveled at the construction and stopped at markers showing the direction of the midsummer sunrise and midwinter sunset. I noticed stone knobs atop some stones like giant primitive Legos while my husband, likewise amazed, joked about aliens.
The day was cloudy, so we didn’t see any stream of light lining up with the remaining stones—and I wasn’t willing to return a few days later and deal with 10,000 other visitors on December 21st—but it was clear that light was very important to the late Neolithic people of the region (around 2500 BCE). In fact, light has been so important for the survival and morale of humanity that we still imbue it with holiness or reverence, perhaps rightly so.
But the light we see is only one part of the full story of electromagnetic radiation, just as the group of remaining stones is only one part of the lengthy, messy history of Stonehenge. Our eyes have evolved to see wavelengths of light 400 to 700 nanometers long, where one nanometer is 1/1,000,000,000 of a meter or 10-9 meters. But the full spectrum of what can still be called light ranges from radio waves—long wavelengths, about 103 meters each—to gamma rays, very short wavelengths of about 10-12 meters each. All the light along this spectrum is “radiation.”
Since light acts as both a wave and a particle, we can also talk about photons, which are little packets of light. I use photons to research cosmic rays with the Telescope Array Project; photons are how we detect a high-energy particle colliding with our atmosphere, like shards of glass left after a baseball breaks a window.
To see light in action, how could we calculate the light energy of just one photon streaming from the sun to the Earth, then through the Earth’s atmosphere at sunset into the center of Stonehenge during the winter solstice? It’s a set of formulas to follow, just as we’d follow a recipe to bake gingerbread cookies or make mulled wine.
- First, we need to know that the relationship wavelength × frequency = speed holds for any wave. Wavelength is a length, and frequency is “cycles per amount of time,” so together they give us length divided by time, the definition of speed. The speed of light—we use the variable c—is always constant in the vacuum of space, as you probably remember from school. In the cold winter air, light slows down slightly, but since the difference is so small, we’ll use the standard c = 3.0 × 108 meters per second (m/s) for the light traveling at sunset from the sun to the center of Stonehenge.
- Second, sunlight peaks roughly in the green part of the visible spectrum, which means the light’s average wavelength (as denoted by λ, the Greek letter lambda) is around 550 nanometers. Wavelength would be slightly shorter in the cold winter air, but again, it’s a slight difference. Since we know wavelength and the speed of light, we can now calculate the frequency of solstice sunlight to be 5.45 × 1014 hertz (for more on frequency and its units of hertz, see Citizen Science No. 19).
- Finally, the radiative or light energy E (measured in joules!) carried by one photon of light follows the recipe E = h × f, where f is the frequency we already found and h is Planck’s constant, h = 6.626 × 10-34 joules per hertz. Planck’s constant is like the tiny 1/8 teaspoon you use for ground cloves in Christmas cookies: It sets the smallest effective amount of an ingredient (in this case, energy) that can be added to the system. With this recipe, we now see that one photon streaming from the midwinter sunset into Stonehenge has an energy of 3.61 × 10-19 joules. (This is, less mystically, the exact same energy as the photons guiding my way around the site even on a cloudy day.) While one photon by itself doesn’t do much, many photons together can heat your skin or light your way in the darkness, just as many people together built Stonehenge to honor the light that guided their lives, seasons, memorials and celebrations throughout the turning year.
Like driving on the left side of the road, we’ve given our brains a workout this year in Citizen Science, covering a different kind of energy each month. You can find links to all 12 on AllOtsego.com. Each energy is one face of a many-sided dice: They are all ways to measure the ability to cause change or perform work. They convert to each other such that the energy is always conserved. They are always measured in joules or an equivalent unit. This real, measurable energy is more fascinating and useful to me than any wishful, mysterious, pseudoscientific rubbish.
In the Stonehenge museum exhibit afterward, we learned that late Neolithic inhabitants had access to rope and wood and chipping tools so they could haul stones, shape them—with knobs and notches to connect them—and hoist them aloft. No aliens or mystical energy needed, just hard work—the transfer of one kind of energy to another—plus impressive cooperation and dedication to figuring out, as best they could, the universe they lived in. No matter what religious beliefs we may or may not hold, it is lovely to honor the light that sustains us all. Happy holidays and thanks for reading.
Jamie Zvirzdin researches cosmic rays with the Telescope Array Project, teaches science writing at Johns Hopkins University and is the author of “Subatomic Writing.”